Genomics: Insight
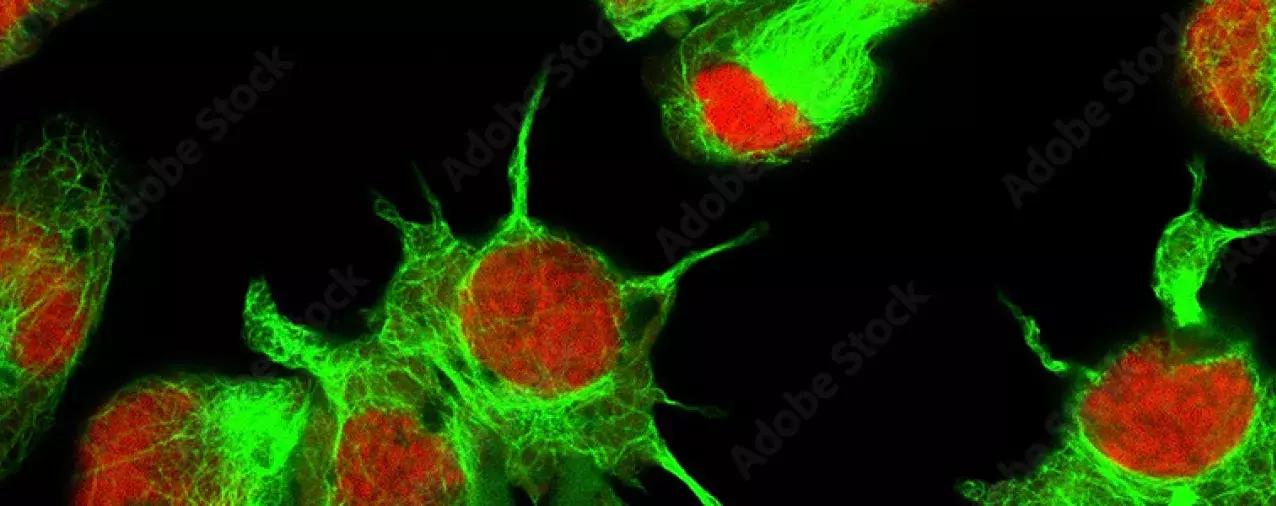
CRISPR-Cas9: a feasible tool to combat neuroblastoma?
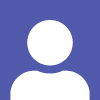
Research Question: How can CRISPR-Cas9 be used to combat neuroblastoma?
Introduction
Neuroblastoma is a malignant form of cancer which begins in the neuroblasts (immature nerve cells). It typically occurs in the adrenal glands, but can also occur in the chest, abdomen, or spine . It often affects young children; it has an incidence rate of “11 to 13 per million children aged younger than 15 years old”.2
In severe cases, the 5-year survival rate is as low as 50% . This is a horrible problem, which needs a solution. CRISPR-Cas9, a genome editing tool could provide this. With CRISPR-Cas9, it is possible to conduct a deletion of certain genes which have proved to be detrimental in causing rapid tumor growth. Throughout this article, we will discuss the feasibility and usage of CRISPR-Cas9 in enhancing the understanding of neuroblastoma’s biological mechanisms as well as providing possible treatments.
"Throughout this article, we will discuss the feasibility and usage of CRISPR-Cas9 in enhancing the understanding of neuroblastoma’s biological mechanisms as well as providing possible treatments."
Understanding the key cellular pathways driving neuroblastoma with CRISPR-Cas9
CRISPR-Cas9 has proved to be an invaluable tool to combat neuroblastoma by providing insight into the expression of certain genes within cancerous cells through in vivo and in vitro knockout screening. This is an instrumental step forwards in our understanding of neuroblastoma, paving the way for specific gene-targeted to fight the growth of neuroblastoma tumors.
Neuroblastoma cells display irregular expression of several epigenetic and transcriptional (EP-TF) genes ; a study in 20244 revealed 27 key EP-TF genes expressed in neuroblastomas, one of which, MYCN, was identified as an upstream regulator of other EP-TF genes, affecting their expression via chromatin remodeling. As well as this, the MYCN gene plays a crucial role in the cell cycle, downregulating CDKN18 and CDKN1A genes, which inhibit cell cycle progression, and upregulating cyclin-dependent kinases and cyclins, promoting cell growth5. The significance of MYCN in driving the progression of tumor growth in neuroblastoma highlights it as a possible therapeutic target. As a transcription factor however, it is difficult to target, due to its lack of structured binding sites. A possible way to reduce MYCN expression is suggested in the paper however. Through a cascade of phosphorylation involving RAS RAF and MEK proteins6, the mitogen-activated protein kinase pathway activates MYCN; by using MEK inhibitors block this pathway, the expression of MYCN can be reduced, decreasing the viability of tumor cells7. Nevertheless, there were some limitations to this study: the primary data came from cells used were sourced from a limited number of patients (60) for in vitro and grafting experiments in mice. It is possible that the data collected therefore may not line up with that of the wider population of those with neuroblastoma and further studies are required. Additionally, they also analyzed retrospective neuroblastoma datasets. Whilst the selection criteria for these datasets was strict, the nature of retrospective data limits the control the researchers had over variables, highlighting the need for further studies.
CRISPR-Cas9 is a powerful tool to understand neuroblastoma. By identifying key genes that show increased levels of activity in neuroblastoma cells, new treatments, such as MEK inhibitors can be trialed. These identified genes can also be used for patient models, such as the ten-gene model developed in this paper4, aiding the stratification of patients into risk categories, to further tailor treatment.
Using CRISPR-cas9 for finding new drug targets
Another study conducted in 2017 found that the neuroblastomas carrying the MYCN amplification show a substantial dependency on polycomb repressive complex 2 (PRC2) components, such as EZH2.8 The researchers in this paper took neuroblastoma cell lines carrying the MYCN amplification, used CRISPR-Cas9 to knock out genes, and monitored cell growth. Through this screening approach, they showed that MYCN amplification correlated with PRC2 activation, as the knockout (KO) reduced cell growth; killing off large proportions of the tumor, and preventing proliferation. This theory was then further validated through other techniques. Indicating EZH2 as a good drug target to combat neuroblastoma. However, this study was conducted in vivo and in vitro on mouse xenograft models. So, these promising findings may not directly translate to human patients.
Combining CRISPR-Cas9 and immunotherapy to fight neuroblastoma
Immunotherapy, is becoming a highly prominent cancer therapy. Making use of the body’s various immune responses to combat cancer, by boosting their ability to recognize and attack cancerous cells. Immunotherapy was a treatment previously ineffective against neuroblastoma, due to its unique tumor immune microenvironment9, which makes it difficult for the body’s immune response to combat; however, with CRISPR-Cas9 this is no longer the case. By using CRISPR-Cas9, you can modify the immune system genes and cell surface enzymes to vary the antigens. In a journal researching the ERAP1 enzyme, CRISPR-Cas9 was used to KO the gene encoding the ERAP1 enzyme in vitro and in mouse xenograft models. This resulted in the surface antigens on the tumor varying, increasing the potent anti-tumor response due to the mass secretion of inflammatory cytokines, improving the recall and activation of CD4+, CD8+ and NK Cells10. The greater response caused a reduction in tumor growth, in vivo, showing the ability for CRISPR-Cas9 to enhance immunotherapy. In another study, CRISPR-Cas9 was used to knock out the TGFβR type II gene in T-cells in vivo and in vitro, and the effects were monitored through various genomic techniques. TGFβ is a pleiotropic cytokine, effectively suppressing the immune system. It can be secreted by neuroblastoma cells. This study found that by genetically modifying CAR T-cells to KO the TGFβRII gene11, they could be made more effective at killing tumor cells than wild type CAR-T cells, due to their ability to resist the anti-proliferative effects from the tumor. This is likely due to the TGFβ cytokine being unable to bind to the surface receptors on the modified CAR-T cells and act as an immunosuppressant, inhibiting its ability to kill tumors. So, by no longer having the TGFβRII gene, these modified CAR-T cells are more effective at removing the tumor. However, this experiment has its limitations, as it does not test the potency of these genetically modified CAR-T cells against neuroblastoma cells, which usually expressed lower levels of tumor-associated antigens, which make it harder for the T-cells to recognize it as foreign and attack.
Although CRISPR-Cas9 is a powerful tool for enhancing immunotherapy, it has some limitations. Car-T therapy itself is quite difficult, particularly for solid tumors due to the limited tumor infiltration.12 In addition to this, the concerns of the off-target effects of CRISPR, editing the wrong DNA site, resulting in undesired gene editing. Which provides difficulties with the implementation. However, when using CRISPR-Cas9 to enhance CAR-T cell therapy and immunotherapy, there are milder ethical concerns. This is because CRISPR-Cas9 is only used to “train” the immune system to recognize certain targets. So can be seen to have less ethical concerns than other gene therapies. In addition, the potential upside of CRISPR is too great. It is a powerful genome editing tool and has massive upside when considering the enhancements in immunotherapy, modifying immune checkpoints.
Conclusion
The application of CRISPR-Cas9 has the potential to make a large-scale difference in dealing with neuroblastoma. As a tool for exploring the condition it has revealed genetic abnormalities within neuroblastoma cells which provides a gateway for the development of new therapies targeted at the repression or activation of genes, such as the EP-TF genes, that affect the survival of the cancer. This also enables it to aid development of prognostic models for neuroblastoma, which are instrumental in analyzing individual cases and determining personalized treatments. As a therapeutic tool it also shows promise. The results of its use in screening genes help to develop new drug targets within cells to reduce tumor proliferation. On the other hand, this technology can be used to alter the human immune system by modifying surface antigens on tumor cells or editing CAR-T cells to more effectively fight neuroblastoma. In practice, there are challenges applying this technology to wider patients, there has been a lack of clinical trials so the effectiveness of this technology on humans is hard to gauge, there are also ethical concerns raised about the modification of human genes which may lead to some resistance from the public towards the use of CRISPR-Cas9 on patients. Finally, the current costs of full genetic therapy from CRISPR-Cas9 is estimated to be up to £2,000,000 per patient13 in some cases, this is a substantial cost which would certainly hinder the implementation of CRISPR-Cas9 therapies. Despite this, the benefits to patients provided by this technology are huge, if further clinical trials are able to confirm the safe use of CRISPR-Cas9 therapies in vivo, then the upsides look immense for fighting neuroblastoma.
References
- Neuroblastoma (2025) Mayo Clinic. Available at: https://www.mayoclinic.org/diseases-conditions/neuroblastoma/symptoms-causes/syc-20351017 (Accessed: February 2025).
- Yan, P. et al. (2020) ‘Comparison of incidence and outcomes of neuroblastoma in children, adolescents, and adults in the United States: A Surveillance, epidemiology, and end results (SEER) program population study’, Medical Science Monitor, 26. doi:10.12659/msm.927218
- Neuroblastoma survival rates and factors influencing outlook (no date) Healthline. Available at: https://www.healthline.com/health/cancer/neuroblastoma-survival-rate#risk-groups (Accessed: February 2025)
- Zhang, L. et al. (2024) CRISPR-Cas9 screening develops an epigenetic and transcriptional gene signature for risk stratification and target prediction in neuroblastoma, Frontiers. Available at: https://www.frontiersin.org/journals/cell-and-developmental-biology/articles/10.3389/fcell.2024.1433008/full (Accessed: 03 March 2025).
- Gherardi, S. et al. (2013) MYCN-mediated transcriptional repression in neuroblastoma: The other side of the coin, Frontiers in oncology. Available at: https://pmc.ncbi.nlm.nih.gov/articles/PMC3593680/ (Accessed: 01 March 2025).
- Cargnello , M. and Roux, P.P. (2000) Activation and Function of the MAPKs and Their Substrates, the MAPK-Activated Protein Kinases, ASM Journals. Available at: https://journals.asm.org/doi/full/10.1128/mmbr.00031-10?form=MG0AV3 (Accessed: 22 March 2025).
- Huang, M. and Weiss, W.A. (2013a) Neuroblastoma and mycn, Cold Spring Harbor perspectives in medicine. Available at: https://pmc.ncbi.nlm.nih.gov/articles/PMC3784814/#:~:text=Knockdown%20of%20MYCN%20expression%20via,driven%20neuroblastoma%20(Burkhart%20et%20al. (Accessed: 22 March 2025).
- Chen, L. et al. (2017) ‘CRISPR-Cas9 screen reveals a mycn-amplified neuroblastoma dependency on EZH2’, Journal of Clinical Investigation, 128(1), pp. 446–462. doi:10.1172/jci90793
- King, E., Struck, R. and Piskareva, O. (2025) ‘The Triad in current neuroblastoma challenges: Targeting antigens, enhancing effective cytotoxicity and accurate 3D in vitro modelling’, Translational Oncology, 51, p. 102176. doi:10.1016/j.tranon.2024.102176.
- Tempora, P. et al. (2024) ‘Combining ERAP1 silencing and entinostat therapy to overcome resistance to cancer immunotherapy in neuroblastoma’, Journal of Experimental & Clinical Cancer Research, 43(1). doi:10.1186/s13046-024-03180-y.
- Alishah, K. et al. (2021) ‘CRISPR/Cas9-mediated TGFβRII disruption enhances anti-tumor efficacy of human chimeric antigen receptor T cells in vitro’, Journal of Translational Medicine, 19(1). doi:10.1186/s12967-021-03146-0.
- Sterner, R.C. and Sterner, R.M. (2021) ‘Car-T cell therapy: Current limitations and potential strategies’, Blood Cancer Journal, 11(4). doi:10.1038/s41408-021-00459-7.
- Rueda, J., de Miguel Beriain, I. and Montoliu, L. (2024) Affordable pricing of CRISPR treatments is a pressing ethical imperative, The CRISPR journal. Available at: https://pubmed.ncbi.nlm.nih.gov/39392045/ (Accessed: 22 March 2025).
About the Author
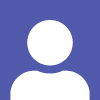
Jaidev Radia: I am an A-level student studying Maths, Biology, and Chemistry at Merchant Taylors’ school, Northwood. I have a particular interest in studying Chemistry or Biochemistry at university. I decided to write this article with my co-author Caspar Haley to further my academic interests and knowledge in the field of biochemistry. Before this, I have given talks on Transition metals, The Ecological and socioeconomic impacts of Kelp Forests, The Biochemistry behind gases used in war, and a paper researching the genomic stability of Sickle Cell Disease. Outside the classroom, I am a talented athlete, playing Rugby and American football at the County level as well representing Team Great Britain, winning an international fixture against Team Spain in American Football.
Caspar Haley: I am an A-level student studying biology, chemistry and english language at Merchant Taylors' School. I have a particular interest in genomics and intent to study biomedical sciences at university. I have previously entered different competitions related to this field, such as the Cambridge Biology Challenge. As a particular interest within genomics, I am highly interested in research, which I am considering a career in.
Mentor: Mrs S Pratt Affiliation: Merchant Taylors' School