Genomics: Insight
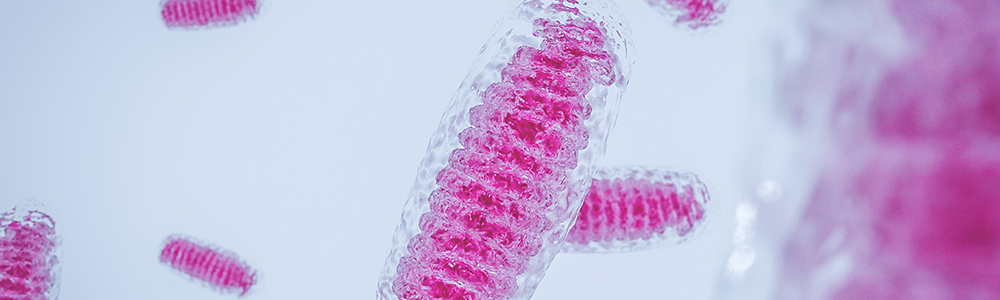
Our Journey to Mastering Mitochondrial DNA
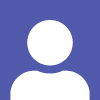
What is DNA?
DNA, a hereditary cornerstone of life, passes and transforms through generations [9]. Specifically, each parent passes a copy of half their chromosomes to their offspring. Genetic information is stored in the form of 4 chemical bases: adenine, guanine, thymine, and cytosine (commonly abbreviated to A, G, T, and C respectively). About 3 million bases that create human DNA are arranged in pairs along sugar and phosphate molecules (known as the sugar-phosphate backbone) to string the iconic double-helix structure. The sequence of such bases determines the construction and regulation of an organism, from eye color to mitosis rate.
While the bulk of DNA resides in our cells’ nuclei (referred to as nuclear DNA), a portion of it can be found in cellular mitochondria (referred to as mtDNA or mitochondrial DNA)[1]. The mitochondrion is an organelle which converts the energy humans receive from food intake into chemical energy that powers cellular systems; the corresponding mtDNA codes for oxidative phosphorylation, a process which releases molecular oxygen to produce ATP (adenosine triphosphate) - an essential energy molecule [6]. Research is still being conducted to determine why cellular power plants have their own genome, but it is widely agreed that mitochondria are the descendants of bacteria that was enveloped, or consumed, by eukaryotes, therefore carrying bacterial qualities including a double membrane system and a separate, self-maintaining genome[1, 6]. Reflective of the varying locations of nuclear and mitochondrial DNA, they aren’t completely alike. Outnumbering nuclear DNA’s 2 copies per cell, the mitochondria house 100-1,000 copies of its genome. mtDNA is circular in contrast to nuclear DNA’s linear structure. The mitochondrial genome contains 16,569 base pairs, a fraction of nuclear DNA’s 3.3 billion base pairs [1, 9]. Relevantly, unlike the heredity pattern of nuclear DNA where each parent makes up half the resulting offspring’s nuclear genome, inheritance of mitochondrial DNA is strictly maternal. While both sexes can inherit mtDNA, it is passed on almost exclusively by females.
Why is mtDNA Significant?
mtDNA is significant because it is primarily transmitted through the female egg [1]. It is also nonrecombinant, meaning it does not combine with any other DNA, remaining unaltered through generations. As a result, one shares their mitochondrial genome with all maternal relatives, including siblings, aunts & uncles, and grandmothers. With increased mutation rates in mtDNA, the mitochondrial sequence may be starkly different among unrelated people, but sequences of maternally related individuals, being greatly similar, are easy to match [1, 6]. One’s mitochondrial genome can effectively be traced back to their first female human ancestor. Broad variations in mtDNA are categorized into what are called haplogroups, which reflect large branch points of humanity’s ancestral - or phylogenetic - tree [2, 3]. The women at the root of these branch points are the common ancestors of all modern humans with their corresponding mitochondrial genome. Defining the evolutionary journey of mtDNA has enabled geneticists to trace matrilineal inheritance of modern humans back to origins in Africa, as well as through our process of globalization. The names of such haplogroups range from A-Z (based on the order of their discovery), and indicate a general timeframe and location of origin. For instance, in terms of origin, the L1-6 haplogroup is predicted to have risen 170,000 years ago in East Africa [2-5]. The U4 haplogroup is estimated to have begun 20,000 years ago in Central Asia. Reflective of our global spread altering geographic distribution, the U4 mitochondrial genome is now most common in modern European populations. Humanity’s ancestral genomic ties, while fascinating, prompt a crucial question: What are the implications of mtDNA?
Defining the evolutionary journey of mtDNA has enabled geneticists to trace matrilineal inheritance of modern humans back to origins in Africa, as well as through our process of globalization.
The Application of mtDNA
Currently, analysis of mtDNA is invoked in various cases. As previously discussed, the establishment of various mtDNA haplogroups paves a clearer path for the deciphering of distant ancestry [2, 3]. Scientists have used such a method to connect modern day human origins to our East African predecessors from 100,000-200,000 years ago. Population geneticists can surface clues about ancient human migration patterns through investigating the prevalence of different haplogroups throughout the world. One such pattern that mtDNA reveals is that early humans who left Africa primarily used one of two paths to Asia (haplogroup M) or to Europe (haplogroup N) [2-5].
Other than for evolutionary purposes, it has historically been used internationally to handle human rights issues [1]. Notably in 1984, Dr. Mary-Claire King - a prominent researcher of human genetics - utilized mitochondrial DNA sequencing to reunite displaced individuals and orphaned children with their families in Argentina.
Similarly, mitochondrial genomes are effective at disproving relationships as much as proving them. If one’s mtDNA is vastly different from their mother’s, it can be concluded that the 2 individuals are not biologically related [1].
Clinically, mitochondrial syndromes are diagnosed through mtDNA testing [1]. Despite millions of mitochondria within the body - each with its own potential for mutations - mothers can harbor mutations that are deemed homoplasmic. This means that all copies of mitochondrial DNA in all of an organism’s eukaryotic cells are identical and harbor a mutation, and all mutations will be transmitted to maternal offspring. Mitochondrial diseases that can be detected include neuropathy, cytopathies, as well as Mitochondrial DNA Depletion syndrome.
Alongside numerous current uses for mitochondrial genome sequencing and analysis, it harbors great potential for future exploration. Broadly, impaired mitochondrial function brings unregulated respiration, insufficient energy production, erratic cellular processes, and increased cell death [1]. Conditions in which mitochondrial deterioration has been associated include incredibly prevalent biological questions, such as cancer, Alzheimer’s disease, and Huntington’s disease. Zooming in on cancer cells, a 1998 study conducted by K. Polyak (internationally recognized oncologist) and her colleagues compared colorectal cancer cells’ mitochondrial genome to that of neighboring healthy colon tissue [1]. 7 out of 10 cell lines investigated showed mutations in mitochondrial genetic material. Consequently, it was hypothesized that the mtDNA mutation may have served as a growth factor - increasing biochemical fuel and division - to cause the uncontrollable cell proliferation that characterizes tumors. Theoretically, mitochondrial genomes may potentially be future targets in attempts to not only slow cell proliferation in cancer, but also to prevent cellular decay that characterizes Huntington’s disease or deterioration of neurons affected by Alzheimer’s.
Theoretically, mitochondrial genomes may potentially be future targets in attempts to not only slow cell proliferation in cancer, but also to prevent cellular decay that characterizes Huntington’s disease or deterioration of neurons affected by Alzheimer's
Summary
Despite having a fraction of nuclear DNA’s 3.3 million bases, mitochondrial DNA is responsible for powering cellular life [6]. Already utilized for various causes, from human rights initiatives to mainstream DNA testing services, mtDNA already brings compelling global contributions. Despite the vastly unknown nature of this fascinating genome and its mechanisms, further investigation has the power to transform our knowledge of history, biomedical research, and clinical treatment for years to come.
About the Author
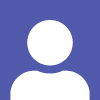
Swetha Chandrasekar is a high school senior at Mundelein High School who hopes to pursue biochemistry and public health in college. Deeply invested in molecular research, epidemiology, and health disparities, she aims to one day become an impactful physician-scientist.